Researchers visualize quantum effects in electron waves
More silent than a fish? A school of them / Anger can be reduced by publishing one's feelings on paper and then erasing them after receiving an insult
Similar to how light can disperse while passing through a pair of slits and create patterns of dispersion, electrons can likewise exhibit interference phenomena. In 1933, Piotr Kapitza and Paul Dirac demonstrated that an electron beam can undergo diffraction when interacting with a stationary light wave, due to the inherent qualities of the particles.
This interaction leads to the emergence of interference phenomena resulting from the wave-like characteristics of the electron beam.
Professor Reinhard Dörner from Goethe University Frankfurt, along with a team of German and Chinese researchers, has successfully utilized the Kapitza-Dirac effect to visually observe the temporal progression of electron waves, also referred to as the quantum mechanical phase of electrons. The researchers have published their findings in the journal Science.
"It was a former doctoral researcher at our institute, Alexander Hartung, who originally constructed the experimental apparatus," says Dörner.
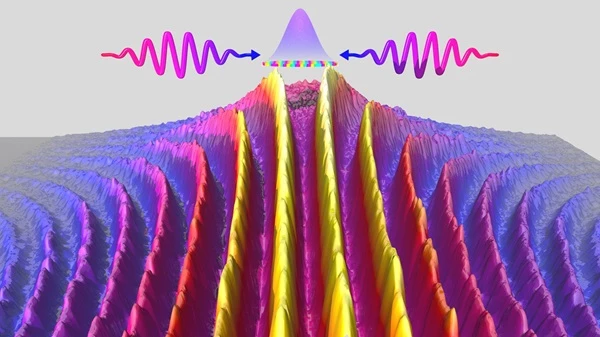
"Following his departure, Kang Lin, a distinguished Alexander von Humboldt fellow who had dedicated 4 years to the Frankfurt team, successfully utilized it to quantify the time-dependent Kapitza-Dirac effect." In order to accomplish this, it was imperative to enhance the theoretical explanation. Kapitza and Dirac, at that time, did not properly account for the temporal progression of the electron phase.
The scientists in Frankfurt initiated their experiment by emitting two ultrashort laser pulses in opposite directions towards a xenon gas.
At the site of intersection, these femtosecond pulses, which have a duration of one quadrillionth of a second, generated a very powerful light field for a short period of time.
This process caused the removal of electrons from the xenon atoms, resulting in their ionization.
Shortly thereafter, the physicists emitted a second set of brief laser pulses towards the electrons that were liberated in this manner. These laser pulses similarly generated a stationary wave at the focal point.
These pulses exhibited a small decrease in strength and did not induce any more ionization.
Nevertheless, they were now capable of engaging with the unbound electrons, which could be detected using a COLTRIMS reaction microscope created in Frankfurt. "At the point of interaction, three things can happen," says Dörner.
"The electron either does not undergo interaction with the light, or it is deflected in either the left or right direction." As per the principles of quantum physics, the combination of these three possibilities results in a specific probability that is represented by the wave function of the electrons. The electron's cloud-like region, where it is most likely to be found with a specific probability, undergoes a collapse into three-dimensional sections.
The temporal evolution of the wave function and its phase is contingent upon the duration between ionization and the occurrence of the second pair of laser pulses.
"This opens up many exciting applications in quantum physics. Hopefully, it will help us to track how electrons transform from quantum particles into completely normal particles within the shortest space of time. We are already planning to use it to find out more about the entanglement between different particles that Einstein called 'spooky'," says Dörner. As is typically the case in science, the repeated testing of well-established hypotheses has proven to be valuable in this situation as well.
Journal Reference: Kang Lin, Sebastian Eckart, Hao Liang, Alexander Hartung, Sina Jacob, Qinying Ji, Lothar Ph. H. Schmidt, Markus S. Schöffler, Till Jahnke, Maksim Kunitski, Reinhard Dörner. Ultrafast Kapitza-Dirac effect. Science, 2024; 383 (6690): 1467 DOI: 10.1126/science.adn1555
End of content
Không có tin nào tiếp theo